Fluid-Structure Interaction Studies of Coronary Artery Disease Biomechanics
Cardiovascular diseases are the leading cause of death worldwide. In the United States, coronary artery disease accounts for 1 in 7 cardiovascular deaths. Cells in the blood and blood vessels are known to respond to alterations of the mechanical forces of their environment, such as blood flow-induced shear stress and tensile strain caused by the dilation and contraction of the blood vessel itself. Computational models of the coronary arteries are used to estimate fluid shear stress and wall tensile strain that are implicated in the development of atherosclerosis. Here we describe the development of two Fluid-Structure Interaction studies of the left anterior descending portion of the coronary artery in COMSOL®. The first is a meso-scale FSI model of a 19mm section of the left coronary artery. The purpose of this study was to couple the macroscopic regime of blood flow in the coronary arteries with the microscopic regime of cellular interactions, in particular endothelial cell-platelet interactions. The 3D geometry of the coronary artery was established using a patient’s CTA data; and a fibrous plaque was inserted into the artery, creating a 70% reduction in vessel diameter, which is clinically classified as a “severe” stenosis. This FSI model was able to provide detailed information in flow patterns, wall shear stress, and wall circumferential strain near or within the plaque with a spatial resolution of 21μm and a temporal resolution of 0.05 s. The average element size in this model was 50μm, which was comparable to vascular endothelial cells (20-100μm or bigger) and circulating platelets (2-4μm), enabling us to observe their interactions driven by blood flow. The second model was a macro-scale coronary artery model with a length of 7.56 cm. This model was also established using a patient-specific geometry (from CTA data). It would be used to assess the spatio-temporal distribution of fluid shear stress and wall tensile strain along the vascular wall and identify mechanical conditions that would promote atherosclerotic plaque growth. The significance of this model include: the patient-specific geometry, large volume (much bigger than most of the reported coronary artery numerical models), transient blood flow, non-linear elastic wall property, and transient squeezing induced by the myocardial contraction. Both of these studies required the use of the CFD and Solid Mechanics Modules and the platelet interaction studies would be performed with the Particle Tracing Module. Since the artery was treated as a hyper-elastic material, the Non-Linear Structural Materials module was employed. The fluid-structure interaction problem will be fully coupled at the interface between the fluid domain, representing the blood volume, and the solid domain, representing the artery wall or plaque material as appropriate. Both studies provided new perspectives on the combined effects of blood flow and vessel strain on the development of cardiovascular disease states.
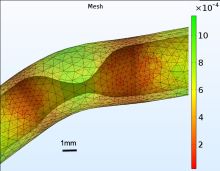