Predicting Arrhenius Parameters for Gas-Phase Decomposition Using a Non-Isothermal Reactor
A well-designed isothermal laminar flow tubular reactor (LFTR) can be used as a source of high-quality kinetic rate data provided conditions are such that the plug flow idealization can be applied to its performance. In practice, meeting the necessary criteria for constant temperature and plug flow in LFTR’s becomes more difficult as reactor size is scaled down particularly when test conditions require very high temperatures and ultra short residence times. Nevertheless, small reactors do offer a significant advantage in terms of safety and operating cost when running kinetic rate studies on highly toxic or expensive materials. In this presentation, we will attempt to show how a reactor simulation using COMSOL Multiphysics® was used to derive kinetic decomposition parameters from sets of experimental recovery data obtained using a small (1.8 mL) non-isothermal LFTR. A series of decomposition tests were conducted on the chemical warfare agent simulant diethyl methyl phosphonate (DEMP). The chemical under test was flash vaporized into a helium stream at 300°C along with a thermally stable internal standard (triphenylphosphine oxide) by means of a commercially available pyrolyzer (CDS Pyroprobe® 5200). The vaporized chemicals then flowed through a non-isothermal quartz LFTR exhibiting a roughly parabolic temperature profile with peak temperatures ranging from 500 to 700°C as determined by detailed thermocouple measurements. Reactor residence time was adjusted by varying the helium flow rate. The gaseous effluent from the reactor containing the surviving reactant, internal standard and breakdown products was collected on a solid sorbent. The various components of interest in the effluent stream were subsequently desorbed in helium, separated, and the recovery fraction of the starting chemical was quantified by GC-MS. A 2D-axisymmetric simulation model of the physical LFTR was developed using COMSOL Multiphysics with the add-on Chemical Reaction Engineering and Optimization Modules. The goal was to find a two parameter Arrhenius expression that provided the best match to a set of experimental reactor recovery data using the Levenburg-Marquart least square algorithm. Experimental data input to the simulation consisted of reactant recovery fractions as a function of four temperature profiles and five flow rate/pressure conditions. Reactant concentrations were very low; therefore, physical properties were based on pure helium gas and transport of dilute species was assumed. Diffusivities for the reactant chemicals in helium as a function of temperature and pressure were estimated using correlations developed by Brokaw (1969). The resulting optimized activation energies and pre-exponential factors for DEMP were good predictors of reactor performance and, were in good agreement with known published theoretical values (Glaude, et.al., 2000).
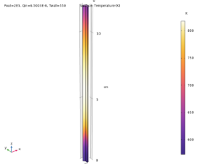