A Parametric Study of Shock Wave Simulations with Help of COMSOL Multiphysics
Adiabatic compression of gases can work as an ignition source and is still one of the main causes of accidents in chemical plants processing tetrafluoroethylene (Reza and Christiansen, 2007). The ignition of tetrafluoroethylene induced by adiabatic compression has been studied experimentally with a setup which allowed for the rapid opening of a high speed valve connecting two portions of a pipeline at different initial pressures (Meyer, 2009). Due to the fast opening time and to the high pressure difference, a shock wave in the pipeline was generated. The propagation of the shock wave and its reflection at the end of the pipeline caused pressure and temperature increase. This led to some ignitions in the experiments performed. Nonetheless, in some test an ignition was not achieved, even if this was expected according to the theoretical temperatures predicted by the Rankine-Hugoniot equations (see Lamnaouer, 2004 or McMillan, 2004 for the equations). In order to understand the discrepancy between the experimental results and the theoretical predictions, shock wave simulations have been carried out with COMSOL Multiphysics. The “High Mach Flow” interface was used, since it solves the heat and impulse equations for fast flows. Figure 1 and Figure 2 show, respectively, the velocity and temperature distribution over time for a simulation in a 0.2 m pipeline of 20 mm in diameter with the following settings: high pressure section: nitrogen initially at 20 bara; low pressure section: nitrogen initially at 1 bara; initial temperature of the system: 20 °C; adiabatic walls with slip condition for the flow; laminar flow. As from Figure 1 and Figure 2 the shock wave generation and propagation has been properly computed and the physical properties of the shock wave reflected the prediction of the Rankine-Hugoniot equation. Nonetheless, divergence problem occurred when trying to add turbulence to the system and strange temperature and profiles after the shock wave reflection were achieved if the no slip condition at the walls was chosen. Despite these limitations, it was possible to perform a parametric study and to analyze the effect of the pipe diameter and length on the shock wave evolution. Here simulations with constant wall temperature were carried out, in order to account for the heat losses to the pipe surroundings. Figure 3 shows that the temperature of the reflected wave is maintained for a longer time, if the pipe diameter is larger, due to minor heat losses. On the other hand, Figure 4 shows that higher average temperatures are achieved and maintained for a longer time if the pipe length increases. These results suggest that in the experiments performed by Meyer (2009) the pipe geometry was probably not optimal for the achievement/conservation of high temperatures and might explain the difficulty in inducing ignitions by adiabatic compression.
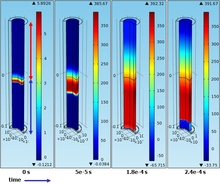
Download
- ferrero_poster.pdf - 0.37MB
- ferrero_paper.pdf - 0.3MB
- ferrero_abstract.pdf - 0.38MB