Simulating the Coupled Mass and Heat Transport in Paperboard During an Induction Sealing Process
Package material for liquid food packages typically features a multilayered structure with paperboard as a core material and protective polymer layers towards the product and the ambient. Aseptic food packages require an additional barrier layer comprising a thin aluminum layer. Aluminum is a light and mass transport barrier and is also an enabler of package sealing through induction heating (IH). Adopting an IH-sealing technique, the package material is exposed to an alternating magnetic field, generating eddy currents in, and consequently heat, the aluminum. By conduction, the heat is transferred to the inside polymer, which melts and, when pressed together, creates a package seal. The time of a seal is limited, typically less than 1 s, and the rapid and substantial heating and compression puts high demands on the packaging material. Using paperboard-based packaging material has several benefits, such as sustainability and a high stiffness-to-weight ratio. However, the hygroscopic nature of paperboard renders the structure sensitive to moisture and high temperatures. Aiming towards a simulation-driven design requires advanced material models in the virtual representation of the packaging material. This work focuses on the coupled mass and energy transport in paperboard. The material model of the paperboard is derived within a Mixture Theory (MT) framework where each Representative Volume Element, (RVE), is viewed as a superposition of three immiscible phases; solid fibers, vicinal water, and air. Allowing for phase transformation between vicinal water and water vapor, the air is viewed as a miscible mixture of two constituents; dry air and water vapor, see Figure 1. At this point, the mechanical response is not considered, and the paperboard's response is dictated by four coupled governing equations: three balance of mass equations and one balance of energy equation. Using COMSOL Multiphysics, transient 2D representations of the governing equations are implemented as coupled General form PDE’s and all constitutive relations are specified either as global functions or as component variables. The resulting coupled heat and mass transport model is used in a simulation to describe the board response during an IH-sealing process. Eddy currents are accounted for by an AC/DC Magnetic Fields interface where the thin aluminum layer is treated as a Transition Boundary Condition; the generated power density is plugged into the balance of energy equation via a boundary Flux/Source feature and heat diffusivity of aluminum is modeled through a boundary Weak Contribution. A simplified energy balance is set up for the polymer layers to incorporate a realistic heat evolution. A subsystem test, resembling the IH-seal, is set up where a thermographic camera is used to log the temperature evolution of the inside polymer. A comparison between the model prediction and the experimental data is presented in Figure 2 and it is concluded that a good agreement is found. To motivate the complexity of the MT-model, an additional comparison is made with a conventional model, viewing the paperboard as a one-component solid, and it is clear that the performance is improved with the MT-model.
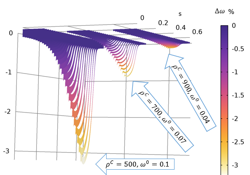